For a PDF of this paper click here
Introduction
Geotech’s latest implementation of its patented airborne AFMAG (Audio Frequency Magnetic) technology is the Z-TEM or Z-Axis Tipper Electromagnetic system. Z-TEM was introduced in mid-2007 and over 20,000 line kms have been flown commercially for minerals exploration. In theory, as with some other electromagnetic techniques, it has applications for petroleum prospecting. From numerical modeling of various hydrocarbon plays, Z-TEM has a depth of investigation for resistive targets to several kilometers.
Z-TEM uses the natural or passive fields of the Earth as the source of transmitted energy. These natural fields are planar and due to the manner in which they propagate, are horizontal. Any vertical EM field is caused by conductivity contrasts in the Earth. The Z-TEM system measures the vertical EM field in the air along the survey lines and makes use of a base station to measure the horizontal fields. The assumption is that the horizontal fields are relatively homogenous over the survey area and the base station location. The vertical EM field is remotely referenced to the horizontal EM fields.
Instrumentation
Z-TEM instrumentation consists of an eight metre single axis air-cored airborne coil measuring the vertical component of the EM field. A suspension system (patent pending) isolates the coil from most vibrations originating from the helicopter and from the air turbulence of the shell during flight. The airborne coil is deployed from a helicopter using a 90 metre long cable to separate the coil as much as possible from the helicopter EM noise while still allowing the pilot to maintain good control of the EM coil.
The attitude of the airborne coil must be monitored in order to remove the cross coupling between the horizontal and vertical EM field. Three GPS receivers are placed on the Z-TEM airborne coil. The data is processed using one of the GPS receivers as a moving base station and calculates the relative positions of the other two receivers to yield a very accurate attitude measurement.
The horizontal reference field is measured by a base station consisting of two, four metre square air-core coils placed orthogonal to each other. The orientation of both units is not critical as the horizontal field can be decomposed into the two orientations of the survey flight. An optional coil is sometimes deployed on the ground to measure the Z component at the base station.
We have conducted experiments on the maximum distance between the airborne coil and the base stations to determine when we have to move the base stations closer to the survey area. At this time we have operated up to 80 kms away from the base station and have still obtain coherent signals between the airborne and base station coils.
Numerical Simulation
In petroleum exploration, the targets are resistive and large as opposed to minerals exploration where smaller conductive targets are sought. Numerical modeling of simple shapes representing, say, salt domes, or oil filled reservoirs, or tar sands were conducted using the EMIGMA modeling package from Petros Eikon. The results of the modeling show that Z-TEM responds well to resistive targets. This is thought to be due to the uniform excitation of the large geological structures by the natural fields.
Figure 2 shows the in-line, inphase Tipper response over a resistive feature representing a salt dome as calculated using the Emigma modeling package. The resistivity of the two kilometer (cylindrical) diameter body is 500 ohm-m embedded in a half-space of 50 ohm-m. The top of the dome is 600 metres below the surface. The source polarization for the model is from the east (in-line direction) and the peak-to-peak value of the ratio is 0.025 which is well above the noise floor of the instrumentation.
We usually assume that the targets are resistive targets, but sometimes a small change in the location of the target in certain geological situations would make the same body a conductive target. Figure 3 shows a 100 ohm-m block situated at the interface of a conductive overburden of 20 ohm-m resistivity over a 200 ohm-m half-space representing the bedrock. The 100 ohm-m could represent a number of petroleum targets such as tar sands, or an oil-filled sandstone channel. If the target was in the bedrock, it is a conductive target. If it was in the overburden, it would be a resistive target.

Figure 3: Two slightly different scenarios of where a “resistive” target is situated at a contact of different resistivities.
Figure 4 shows the Z-TEM response over the two slightly different scenarios. Note the change in the anomaly shapes. Also, the amplitude of the resistive target scenario (top) is ten times larger than the conductive scenario (bottom). While the resistive scenario is detectable with Z-TEM, the response of the conductive scenario is below the noise floor of the system.
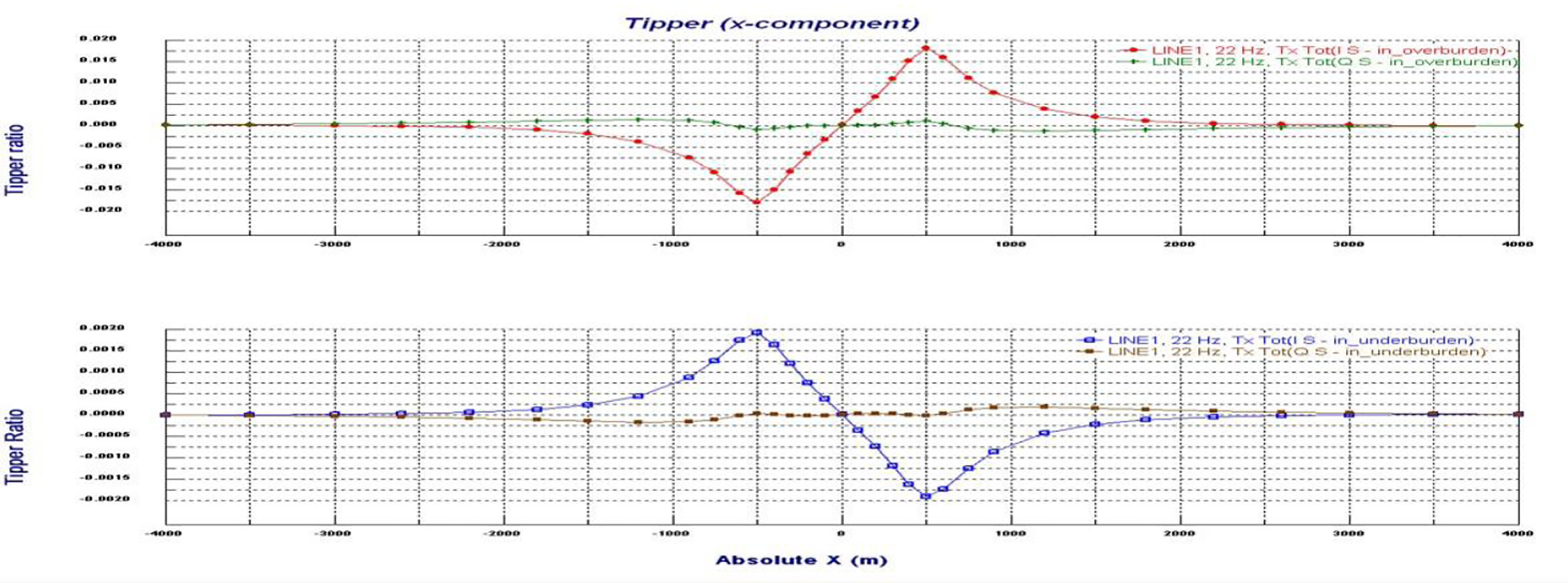
Figure 4: Z-TEM response over the two different scenarios of Figure 3. Top is the resistive target case.
Conclusion
Geotech’s latest implementation of airborne AFMAG has demonstrated its exploration utility in minerals exploration. While at the time of this paper, the Z-TEM system has not conducted a survey for petroleum exploration, numerical studies show that petroleum plays can be detected as a resistivity anomaly. Numerical modeling and good understanding of the resistivities of the targets and host, and of various scenarios will reduce the risk of the Z-TEM survey for petroleum.
Acknowledgements
The authors would like to thank Airborne Petroleum Geophysics and Geotech for permission to publish and present this paper. Ross Groom of Petros Eikon gave valuable feedback on MT theory and processing.
References
Kuzmin, P., Lo, B., Morrison, E., Final Report on Modeling, interpretation methods and field trials of an existing prototype AFMAG system, Misc. Data Release 167, Ontario Geological Survey, 2005.