Presented at SEG 2012
For a PDF of this paper click here
Summary
The 10 year development in airborne passive EM technology originally started in 2000, with a receiver design that combined earlier and new system technologies, as well as advanced digital signal processing used in modern MT. This culminated in a lightweight digital AFMAG towed-bird receiver in 2001. Subsequent improvements led to a relatively successful prototype, in 2002. The addition of a base-station in 2004 improved the signal-to-noise and receiver weight, spurring the development of the ZTEM system in 2005, the first successful and commercially available airborne AFMAG system in 30 years. Since then new technologies include the innovative AirMt system in 2009, a Fixed-wing ZTEM system in 2011, and improved mobility in base-station design in 2012.
Introduction
It has been over 10 years since Geotech Ltd. (Aurora, CAN) first revisited airborne AFMAG (Audio Frequency Magnetics) in 2000, using digital technology and modern signal processing tools (Kuzmin et al., 2005), and more than five years since introducing the first passive airborne EM (AEM) survey results at AESC in Melbourne AU in 2006 (Lo et al., 2006). In the period since then, it developed the ZTEM (Z-axis Tipper Electromagnetic) system in 2006 the first and only successful, commercially available passive airborne AFMAG EM system of its kind in mineral exploration in more than 30 years. Since then, it remains the lone supplier in airborne AFMAG instrumentation, surveying, processing and interpretation, with significant advances being made to its systems for improved electrical imaging for regional reconnaissance resistivity mapping in mineral exploration – notably the inception of the AirMt (Airborne Magnetic Tensor) system in 2009 and, most recently, its Fixed Wing ZTEM system in 2011.
General Theory
In airborne or ground AFMAG (Ward, 1959; Labson et al., 1985), the horizontal and vertical magnetic field transfer functions are related by the following:
whereby WZX and WZY form a complex vector known as the tipper or induction vector, also called the Weiss-Parkinson vector. The vertical magnetic field is zero for plane waves vertically propagating into a 1D earth model, non-zero vertical magnetic fields are directly related to 2D or 3D structures (Gribenko et al., 2011).
ZTEM (Lo and Zang, 2008) is the airborne variant of AFMAG that measures the tipper components as the transfer function of a vertical magnetic field measured from an airborne receiver to the horizontal components measured at a ground-based reference receiver:
AirMt (Kaminski et al, 2010), a closely related airborne AFMAG technique, directly measures the rotational invariant of the transfer function for the three magnetic fields measured from an airborne receiver to those from a ground-based (reference) location. The three components of a magnetic field measured at a receiver are linearly related to the magnetic fields measured at a ground-based reference receiver, according to:
W1 and W2 can be written as the first and second columns of the transfer function, then we can introduce the variable: , obtaining the complex scalar:
called the amplification parameter (AP), which can be shown to be rotationally invariant (Kuzmin et al., 2010; Dodds, 2010, pers. comms.; Wannamaker, 2010, pers. comms.). Since the amplification parameter does not depend on the orientation of the sensor, it is negates the post-acquisition need to correct for sensor orientation (Legault et al., 2012).
Passive AEM System Development
AFMAG systems all measure the anomalous vertical secondary magnetic fields that are created by the interaction between electrical heterogeneities in the earth and naturally occurring, plane wave audio frequency EM fields that are from worldwide thunderstorm activity – the same as those used in audio-magnetotellurics (AMT), known for its great depth of penetration, up to several km (Strangway et al., 1973). Airborne AFMAG is therefore a unique airborne deep resistivity mapping tool capable of exceeding the depth penetration limits typically expected from traditional airborne EM technologies.
The early airborne AFMAG development in 2000 focused on a towed-bird, lightweight digital receiver (Figure 1), whose acquisition system was based on the earlier Hawk broadband fixed wing-tip frequency-domain and the new VTEM 24-bit time-domain digital acquisition systems (Kuzmin et al., 2005). By 2001, it had been determined that sensor size and vibrational motion noise were significant factors. Subsequent designs used a slightly larger, more sensitive multi-coil receiver (Figure 2) with damping mechanisms and attitude sensors, operating in the 20-6000Hz frequency band. A base-reference station was also included to record individual fields for remote-referencing and also to permit the use of single coil to measure the vertical Z-field on the aircraft (Lo and Kuzmin, 2004).
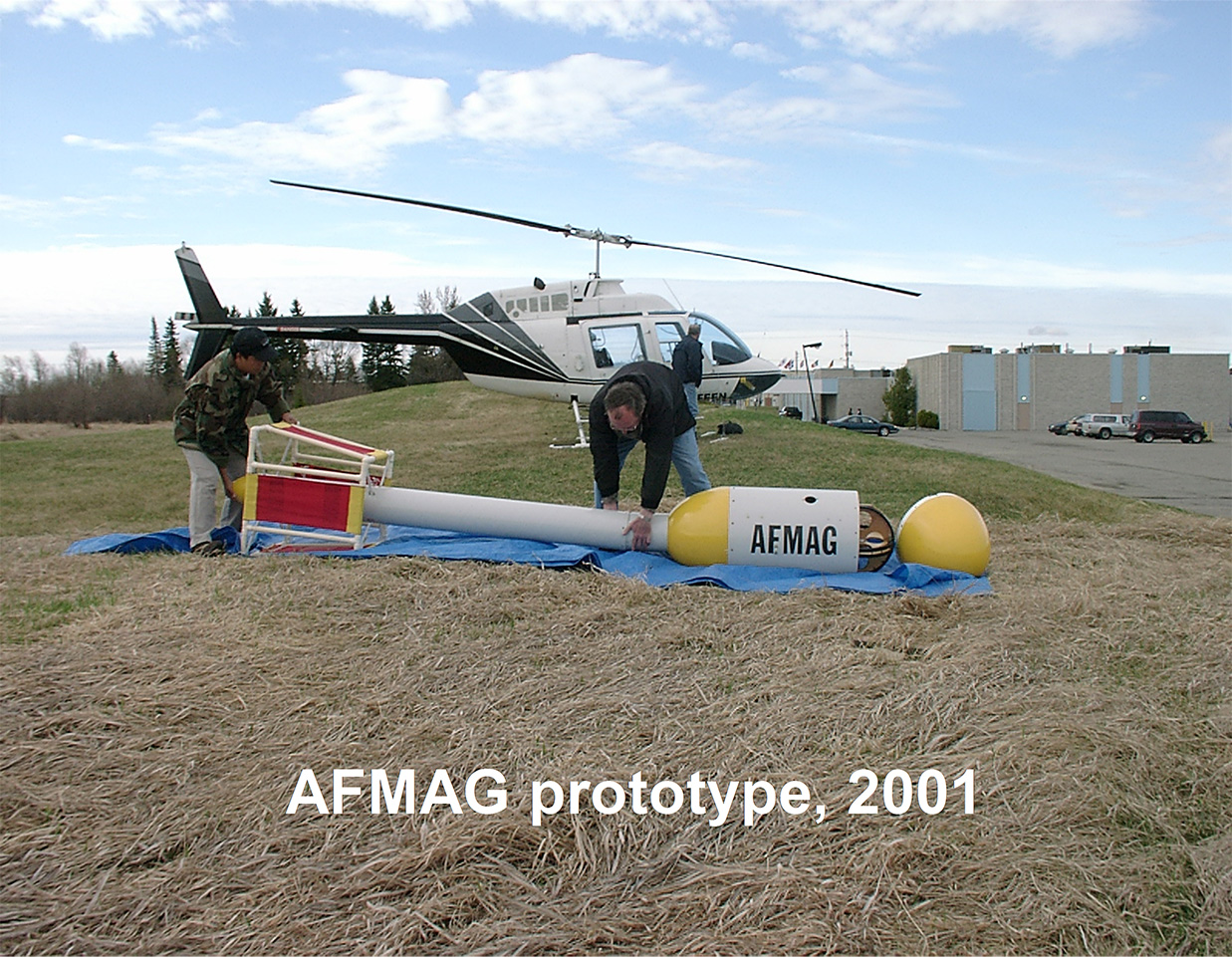
Figure 1: Airborne AFMAG prototype, in early 2001, with nose-cone removed (after Kuzmin et al., 2005).
In addition to hardware development and field testing, this Ontario government-sponsored project also included software development for advanced digital signal processing of time-series, similar to that used in ground magnetotelluric soundings (Anav et al., 1976;) and ground tippers, as proposed by Labson et al. (1985); as well as attitude corrections and noise rejection (Kuzmin et al., 2005).
The AFMAG system development culminated in relatively successful field trials in 2002 at the Reid Mahaffy test site (Witherly et al., 2004) and in 2002 and 2004 in the Sudbury area in northern Ontario, Canada, as well as fixed-wing tests, in 2002, that were described by Kuzmin et al. (2005), Lo et al. (2005) and Lo et al., (2008). The studies revealed that the helicopter AFMAG system was workable and the data were repeatable and responded to known conductors. But the tipper data were also noisy and the system required improvements in suspension, better motion noise rejection and larger coils. Tests also showed that a fixed base-station could be used in place of horizontal coils on the receiver, offering a 10x improvement in signal to noise and reducing the weight of the system (Lo and Kuzmin, 2005; Lo et al., 2006). The lessons learned from the AFMAG system testing spawned the ZTEM (Z-axis tipper electromagnetic) helicopter system (Figures 3 and 5).
The ZTEM development, that started in 2005, led to field testing in 2006 (Thompson et al., 2007) and eventually it was fully commercial by 2007 (Lo and Zang, 2008). In ZTEM, only the vertical (Hz) component data are acquired at the receiver, with the horizontal fields (Hx, Hy) obtained at a fixed, stable base-station – the assumption being that the AFMAG primary fields are relatively homogeneous over the survey area and below the base station. The ZTEM receiver is a 7.4 diameter single-axis air-coil that is towed as a sling-load 90m below the aircraft and flown 50-100m nominally above the ground (Figure 4). Built of fiberglass and isolated from vibrational noise using a patented suspension system, the airborne coil is designed to fly horizontally. The attitude positioning of the receiver is enabled by 3 GPS on the receiver as well as GPS and radar on-board the helicopter, as shown in Figure 4. From the attitude information the horizontal field detected by the airborne receiver is removed (Lo and Zang, 2008).
The ZTEM development, that started in 2005, led to field testing in 2006 (Thompson et al., 2007) and eventually it was fully commercial by 2007 (Lo and Zang, 2008). In ZTEM, only the vertical (Hz) component data are acquired at the receiver, with the horizontal fields (Hx, Hy) obtained at a fixed, stable base-station – the assumption being that the AFMAG primary fields are relatively homogeneous over the survey area and below the base station. The ZTEM receiver is a 7.4 diameter single-axis air-coil that is towed as a sling-load 90m below the aircraft and flown 50-100m nominally above the ground (Figure 4). Built of fiberglass and isolated from vibrational noise using a patented suspension system, the airborne coil is designed to fly horizontally. The attitude positioning of the receiver is enabled by 3 GPS on the receiver as well as GPS and radar on-board the helicopter, as shown in Figure 4. From the attitude information the horizontal field detected by the airborne receiver is removed (Lo and Zang, 2008).
In 2006, the ZTEM base station coils were initially identical to the airborne sensors (Figures 3) but their size proved impractical and were replaced by smaller (3.2m) perpendicular coils in 2007 (Figure 4). A 3-axis AirMt sensor coil (3.04m) has also been alternately used as a ZTEM base station since 2009 (Figure 7).
The ZTEM data are sampled at 2 kHz and the vertical component data (Hz) are then synchronously merged with the base station data to produce the appropriate real and imaginary tipper transfer functions using standard magnetotelluric digital signal processing. The in-line (Tzx) and cross-line (Tzy) tipper data are then output at 2.5Hz or approximately 10m interval sampling. Attitude corrections and filtering are performed in a post-acquisition processing step. Initially, 25 to 2800Hz bandwidth signals were analyzed (Lo and Kuzmin, 2008) but now 5-6 frequencies of data, in the 30-720Hz or 25- 600Hz band, are derived, based on signal strength (Lo et al., 2009ab).
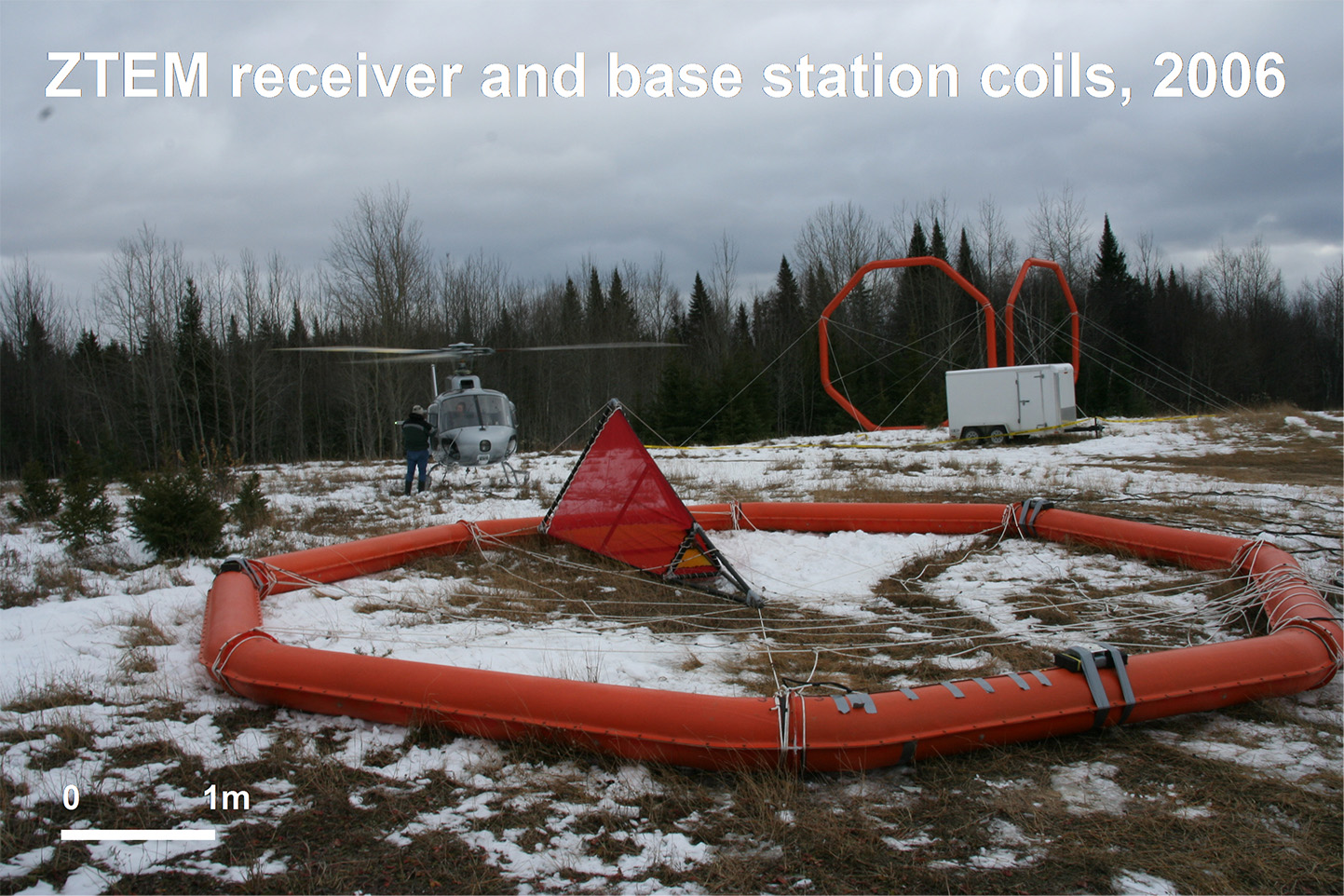
Figure 3: ZTEM z-axis receiver (foreground) and ground-based prototype reference coils (background) from field tests in northern Ontario CAN in 2006 (after Thompson et al., 2007).
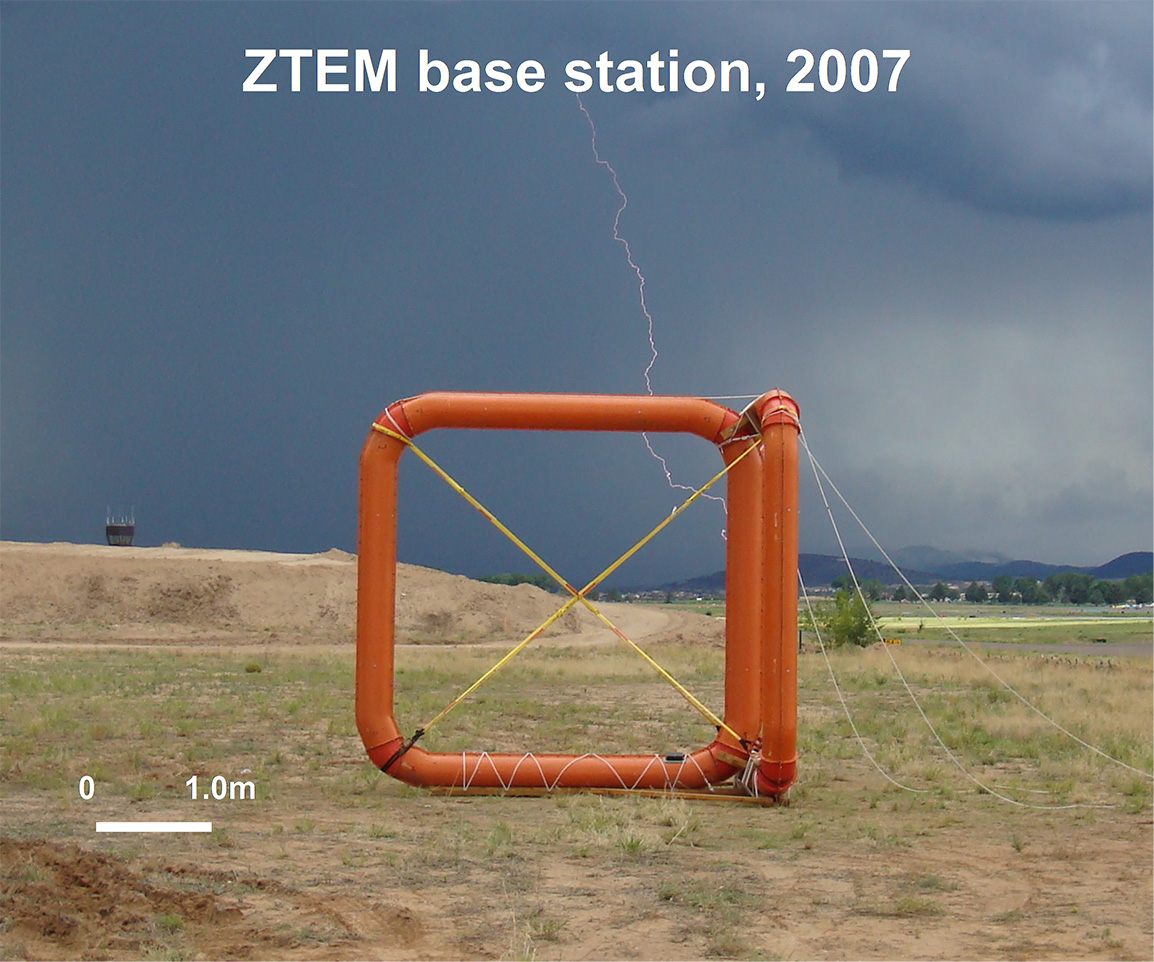
Figure 4: ZTEM base-station receiver in 2007 field surveys in Arizona USA (after Lo and Kuzmin, 2008).
In 2009, the next generation AFMAG system, AirMt (Figure 6), was introduced (Kaminski et al., 2010; Kuzmin et al, 2010). In AirMt all three orthogonal components of primary and secondary magnetic field (Hx, Hy and Hz) are measured at the receiver (Figure 7), as opposed to a single vertical component measurement in ZTEM. The three measured components are further converted into an attitude-invariant tensor amplitude parameter “AP” that effectively eliminates the need for tilt-compensation and therefore significantly improves the signal to noise (and potentially also better depth of penetration) over a standard ZTEM tipper measurement. In-phase and quadrature transfer functions are derived at 4-5 frequencies in the 45-720Hz band(or alternatively 37-600Hz). AirMt is designed to be the next step in Geotech’s AFMAG technology for higher resolution mapping of even deeper geological formations, as well as shallower structures. Currently AirMt is still at an R&D and field testing stage (Legault and Fisk, 2012; Legault et al., 2012) and is not yet commercially available.
The Fixed-Wing (FW) ZTEM system (Legault and Fisk, 2012) is the newest airborne AFMAG development in 2011 and is intended for improved efficiency in more regional geologic mapping applications. Deployed from a Cessna Grand Caravan, the FW-ZTEM uses an identical data acquisition system as the helicopter version but obtains vertical (Hz) component data using a redesigned (3x4m) aircoil receiver, suspended below the fixed-wing aircraft, using a retractable cable, winch and cradle system (Figure 8). The vertical component data (Hz) are also ratioed to fixed horizontal field measurements (Hx-Hy) using smaller, portable ferrite-cored base-station reference coils (Figure 9). In-phase and quadrature transfer functions are derived for the In-line and cross-line tipper at 5-6 frequencies in the 30-720Hz band (or alternatively 25-600Hz).
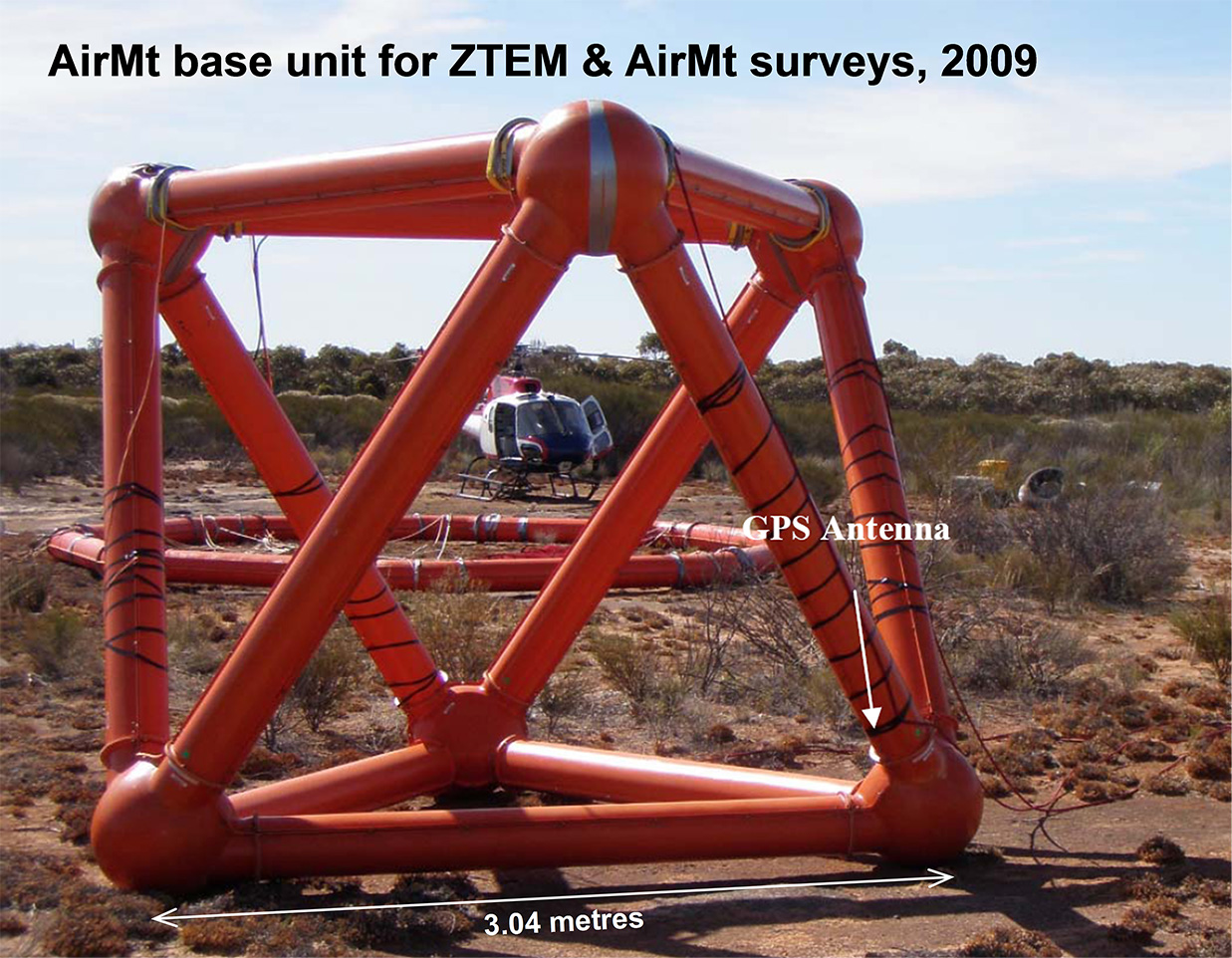
Figure 7: AirMt sensor, deployed as ZTEM and AirMt base-stations, during field trials in Western Australia in 2009.
Currently aeromagnetic data are collected using a tail stinger sensor on the aircraft (Figure 8), but future plans are to place it in the EM receiver assembly. In addition to magnetics and ZTEM, the increased payload in the fixed-wing aircraft would permit adding other sensors, such as gravity/gravity-gradiometry and spectrometry, to the airframe, thereby allowing for the widest possible range of physical property measurements in reconnaissance geologic mapping surveys. Field surveys with the FW-ZTEM system and gravity-gradiometer are planned for 2012.
Conclusions
It has now been more than ten years since embarking on the development of airborne AFMAG technology for mineral exploration, starting with a lightweight prototype in 2001, then a fully damped and attitude-corrected receiver as well as modern digital signal acquisition and processing in 2002, followed by successful field trials that proved the merits of a fixed base-station in 2004, that eventually led to the successful ZTEM concept in 2005, and which later became commercially viable in 2007. This steady progression in technology subsequently led to the AirMt airborne AFMAG system in 2009, continues with the more recent development of the Fixed-wing ZTEM system in 2011, and smaller, more mobile base station sensors in 2012.
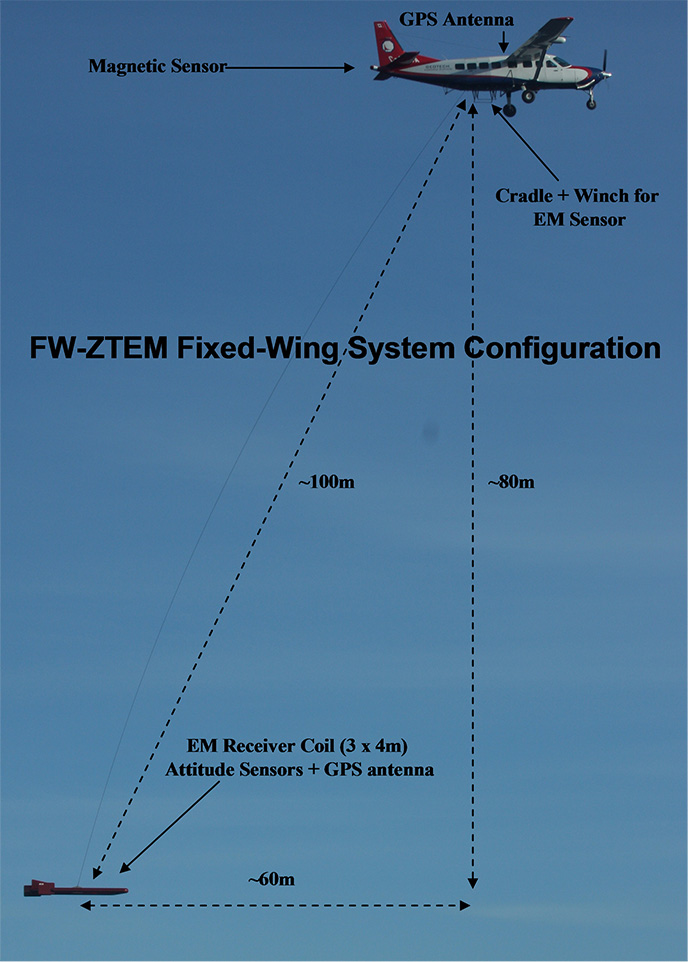
Figure 8: FW ZTEM fixed-wing system aboard Cessna Grand Caravan, during field trials in Arizona USA in 2011.
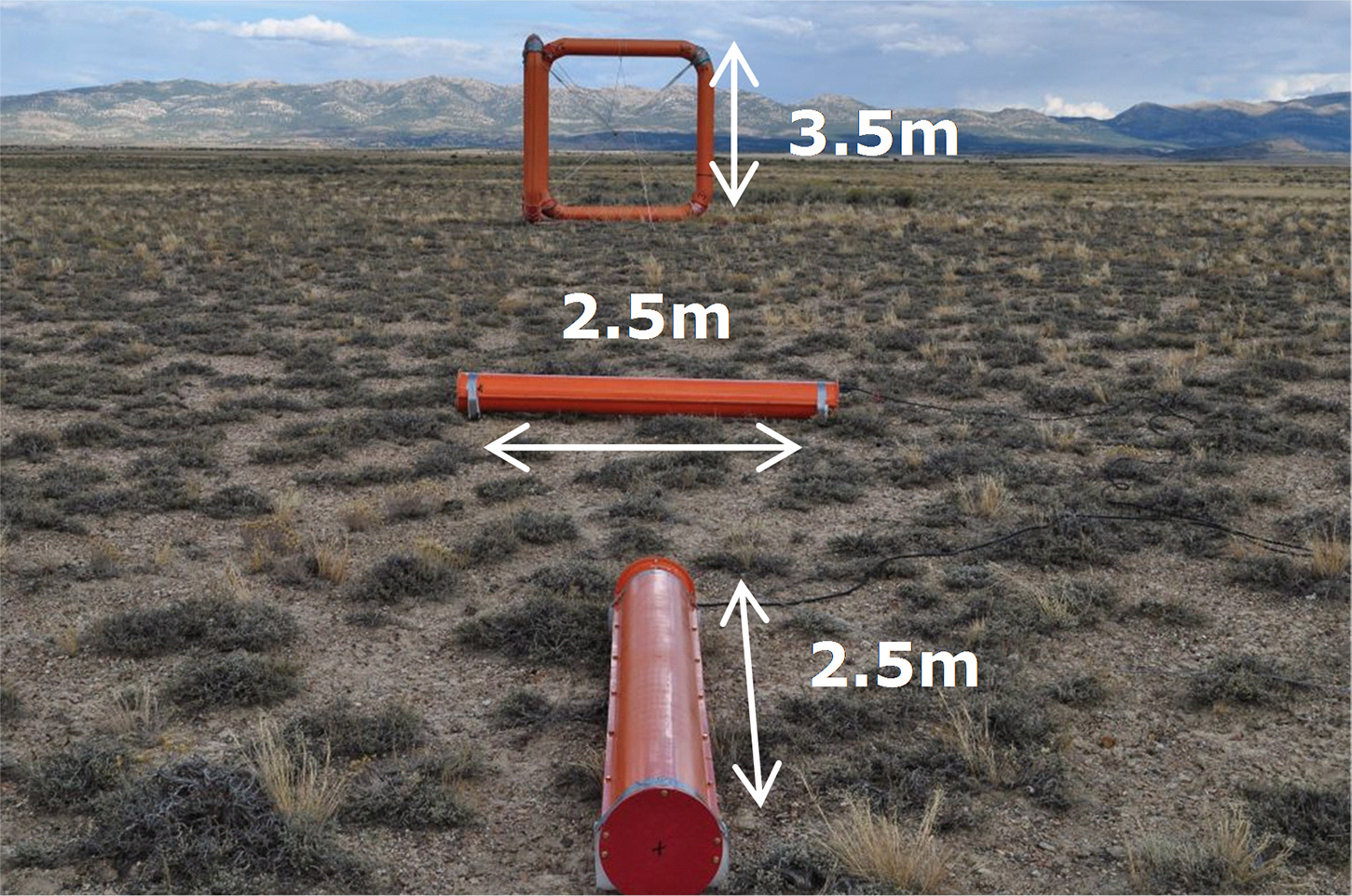
Figure 9: New (foreground) and old (background) ZTEM base-station sensors, during field trials in Nevada USA in 2011.
Acknowledgements
The author gratefully acknowledges Jack Dodds (Geo Manufacturing), Philip Wannamaker (Consultant), Michael Zhdanov (University of Utah) and Glenn Wilson (TechnoImaging) for the theoretical development presented in this paper.
References
Anav, A., S. Cantarano, P. Cerruli-Irelli, and G. V. Pallotino, 1976, A correlation method for measurement of variable magnetic fields: Geoscience of Electricity, GE14, 106–114.
Fountain, D., 2008, 60 years of airborne EM – focus on the last decade: presented at the AEM 2008, 5TH International Conference on Airborne Electromagnetics.
Gribenko, A. V., M. S. Zhdanov, M. Čuma, L. F. Cox, G. A. Wilson, and J. M. Legault, 2011, 3D inversion of ZTEM and AirMt data – A case study from the Nebo-Babel Ni-Cu-PGE deposit, West Musgrave, Western Australia, presented at GEM 2011 international workshop on gravity, electrical & magnetic methods and their applications.
Kaminski, V. F., P. Kuzmin, and J. M. Legault, 2010, AirMt – passive airborne EM system, presented at 3rd CMOS-CGU Congress.
Kuzmin, P., B. Lo, and E. Morrison, 2005, Final report on modeling, interpretation methods and field trials of an existing prototype AFMAG system, for Ontario Mineral Exploration Technology Program (OMET), Project No. P0102-007b, Ontario Ministry of Natural Resources publication MRD-167, 75 pp.
Kuzmin, P. V., G. Borel, E. B. Morrison, E. B. and D. J. Dodds, 2010, Geophysical prospecting using rotationally invariant parameters of natural electromagnetic fields: WIPO International Patent Application No. PCT/CA2009/001865.
Labson, V. F., A. Becker., H. F. Morrison, and U. Conti, 1985, Geophysical exploration with audio frequency natural magnetic fields, Geophysics, 50, 656-664.
Legault, J. M., and K. Fisk, 2012, Statement of Capability – Geotech Airborne Ltd., In R.J.L. Lane (editor), Natural Fields EM Forum 2012: Abstracts from the ASEG Natural Fields EM Forum 2012: Published by Geoscience Australia, Geoscience Australia Record 2012/04, 10-21.
Legault, J.M., G. Wilson, A. Gribenko, M. S. Zhdanov, S. Zhao, and K. Fisk, 2012, An overview of the ZTEM and AirMt systems – A case study from the Nebo-Babel Ni-Cu-PGE deposit, West Musgrave, Western Australia, In R.J.L. Lane (editor), Natural Fields EM Forum 2012: Abstracts from the ASEG Natural Fields EM Forum 2012: Published by Geoscience Australia, Geoscience Australia Record 2012/04, 101-121.
Lo, B., and P. Kuzmin, 2004, AFMAG: Geotech’s new Airborne Audio Frequency Electromagnetic system: Houston Geological Society, 47, No. 3, 59-61.
Lo, B., and P. Kuzmin, 2005, Geotech’s Airborne AFMAG EM System: presented at KEGS Airborne Symposium.
Lo, B., and P. Kuzmin, 2008, Z-TEM (airborne AFMAG) as applied to hydrocarbon prospecting: presented at the AEM 2008, 5th International Conference on Airborne Electromagnetics.
Lo, B., P. Kuzmin, and E. Morrison, 2006, Field Tests of Geotech’s Airborne AFMAG EM System: presented at the AESC 2006 conference.
Lo, B., J. M. Legault, P. Kuzmin, and M. Combrinck, 2009, Z-TEM (Airborne AFMAG) tests over unconformity uranium deposits, 20TH ASEG International Geophysical Conference & Exhibition, Adelaide, AU, Extended Abstracts, 5 pp.
Lo, B., and Zang, M., 2008, Numerical modeling of Z-TEM (airborne AFMAG) responses to guide exploration strategies, SEG Expanded Abstracts, 27, 1098-1101.
Strangway, D.W., Swift Jr., C.M., and Holmer, R.C., 1973, The application of audio-frequency magnetotellurics (AMT) to mineral exploration, Geophysics, 38, 1159-1175.
Thomson, S., Fountain, D., and Watts, T., 2007, Airborne geophysics – evolution and revolution, In “Proceedings of Exploration 07: Fifth Decennial International Conference on Mineral Exploration”, edited by B. Milkereit, 19-37.
Ward, S. H., 1959, AFMAG – Airborne and Ground: Geophysics, 24, 761-787.
Witherly, K., Irvine, R., and Godbout, M., Reid Mahaffy test site, Ontario: an example of benchmarking in airborne geophysics, SEG Expanded Abstracts, 23, 1202-1204.