For a PDF of this paper click here
Introduction
Introducing the Full Waveform VTEM™ system, the most recent technological development by Geotech, designed to achieve fully calibrated time-domain EM decays for better near-surface mapping than was previously possible with earlier VTEM™ systems. The new VTEM™ design uses the streamed half-cycle recording of transmitter and receiver waveforms to obtain a complete system response calibration throughout the entire survey flight that helps to precisely eliminate the effect of the data acquisition system response on the recorded signals. The full waveform technology can be added to the standard VTEM™ PLUS system (Figure 1a) without diminishing its patented and industry leading low noise and deep penetration characteristics.
In addition to improving the system bandwidth and the complete system response calibration, newly developed digital signal processing techniques have been applied that are aimed at reducing the effect of the input transmitter waveform and time-varying injected current using both a parasitic loop capacitance correction and a transmitter drift correction, as well as ideal waveform deconvolution. These implementations have helped improve the accuracy of the measured earth-response particularly in the earliest portions of the off-time EM decay – considerably close to the transmitter current turn-off than the 100us limit allowed for by previous VTEM™ systems.
Results of the full waveform VTEM™ helicopter system testing over the Timiskaming Kimberlite Field, in northeastern Ontario (Figure 1b), have shown a significant improvement in quantitative VTEM™ data at earlier times than previously achieved – allowing up to 45 time-gait measurements, from 0.018 to 9.286ms (ch4-47) after the current turn-off. This has resulted in improvements in the model space that include better definition of near-surface conductivity associated with the weathered kimberlite and excellent correlation with magnetic results.
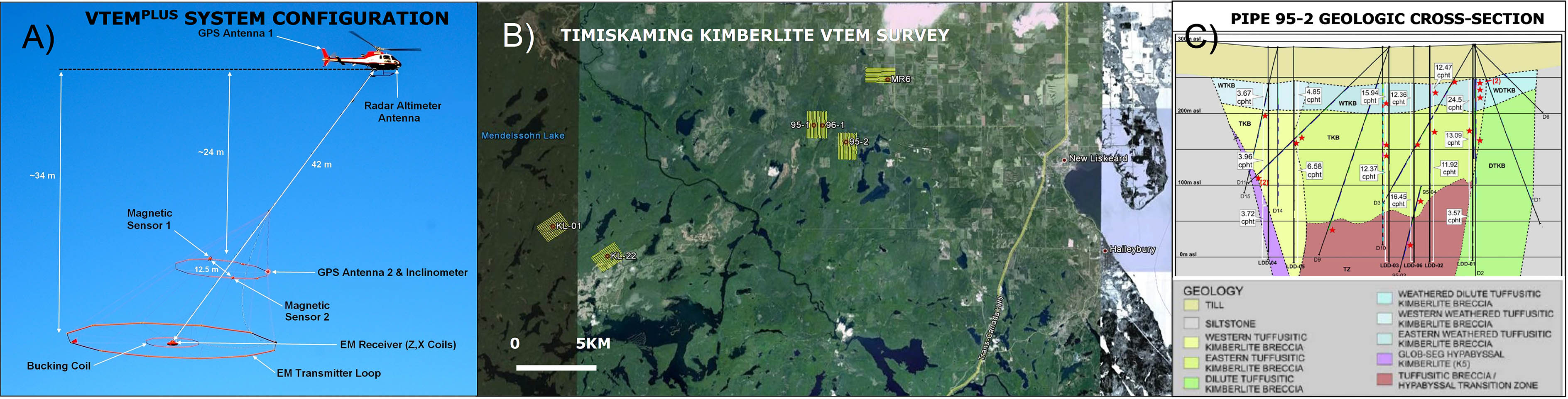
Figure 1a) VTEM™ PLUS helicopter EM system configuration with horizontal magnetic-gradiometer 1b) Timiskaming kimberlite VTEM™ survey locations, showing flight lines and kimberlite (red circles) 1c) Geologic cross-section across 95-2 kimberlite (courtesy Stornoway Diamond Corporation).
Full Waveform Description
The full waveform VTEM™ system was developed in late 2011 for improved early time data using either standard VTEM™ PLUS or the VTEM™ early time systems. The significant features of the full waveform technology are: a) streamed half-cycle recording of transmitter and receiver waveform data, and, during the post-processing stage, b) continuous system response calibration, c) transmitter drift & parasitic noise correction, and d) ideal waveform deconvolution.
The sensor calibration procedure uses the measured calibration waveform for correction of half-cycle waveforms acquired on a survey flight. The half-cycle waveforms of each channel are corrected to obtain the waveforms that would be recorded if the time-domain responses of all the channels, including the reference channel, were the same ideal Gaussian-like response. The ideal response is defined by its bandwidth.
A streamed current monitor and streamed receiver data are used for transmitter drift and parasitic noise corrections and ideal waveform deconvolution. The deconvolution procedure corrects one complete period for linear system imperfections including transmitter current drift through the following operation:
R(t) ó R(w) = [C0 (ω) B(ω) ] / [C0 (ω) H0 (ω) W(w)]
Where R(t) is the desired response (corresponding to R(w) in frequency domain), C is the instantaneous current monitor and C0 the averaged high altitude reference current monitor measurement, B the instantaneous survey data and H0 the averaged high-altitude data respectively. W(w) is an ideal waveform for which the response is desired (Macnae and Baron-Hay, 2010).
Full Waveform Test Results
During early winter, 2011, Geotech conducted a helicopter-borne geophysical survey over the Timiskaming Kimberlite Field that belongs to Stornoway Diamond Corporation and is located 15-40km southwest of Earlton and west of New Liskeard-Haileybury, Ontario (Fig. 1b). The field consists of six (6) kimberlite pipe diatremes (95-1, 95-2, (6-1, MR6, KL-01 & KL-22) that are hosted in Proterozoic sediments that are made up of siltstones, argillites, sandstones and conglomerates. The geophysical surveys consisted of helicopter borne EM using the versatile time-domain electromagnetic (VTEM™ PLUS) system with Z and X component measurements and horizontal magnetic gradiometer using two caesium magnetometers. The survey was divided into five (5) blocks (Fig. 1b), with 25-30km flown per
block. The flight lines were generally 10km long, 100m-spaced and oriented in NS, EW or NE directions,. The nominal survey speed was 80kph with a nominal EM bird clearance of 42m and a magnetic sensor clearance of 61 metres. A total of 145.5km were acquired
The VTEM™ survey results were initially processed using standard methods, with the system calibration correction and the parasitic-noise/transmitter-drift/ideal-waveform-deconvolution corrections applied in a separate post-processing step using the streamed full-waveform data. The VTEM™ data were then treated with 1D laterally constrained-inversions (LCI) using an in-house developed heuristic laterally constrained inversion (HLCI) that is based on the AirBeo layered earth 1D TEM inversion code (2007, CSIRO/AMIRA).
Pipe 95-2: The 95-2 kimberlite pipe (Fig. 1c) is overlain by 30 to 50 metres of overburden. The top 20-30 metres of the pipe contains a cap of weathered tuffusitic kimberlite breccia (WTKB). Below the cap lies the tuffusitic kimberlite breccia (TKB). At depth, a hypabyssal transitional zone has been intersected but not fully explored. 95-2 extends approximately 400 metres in an east-west direction and 80-150 metres from north to south. A geological plan view outline of the extent of 95-2 can be seen in Figure 2a. Kimberlites are reflected in the EM data with increased response mostly in early times, from ~120 μsec towards 20 μsec (Fig. 2b & 2d). According to the resistivity depth image (RDI) transforms, inversion and plate modeling (Fig.2cdf), the response is due to the weathered cap of the kimberlite (WTKB).
The primary target of EM data modeling is the weathered cap of kimberlite pipes since it is the most conductive part. EM data models can determine the depth, thickness, conductance and lateral extent of the weathered cap. Resistivity-depth images (RDI), seen in Figure 2f, are good at quickly approximating the depth and lateral extent of the kimberlite pipe. Plate modeling will yield more accurate results for depth and conductance. The plate model for 95-2, shown in blue of Figure 2f, is horizontal and at a depth of 130 metres. This depth puts the modeled plate precisely in the middle of the weathered kimberlite layer. The EM response profiles showing the measured and modeled response are shown in Figure 3D. The LCI is able to determine the approximate depth, thickness and extent of the weathered cap. Figure 2c shows a LCI for 95-2 with an approximate depth of 100 metres and thickness of 30 metres. The LCI results have the potential to map the top and bottom of the weathered cap of kimberlite pipes.
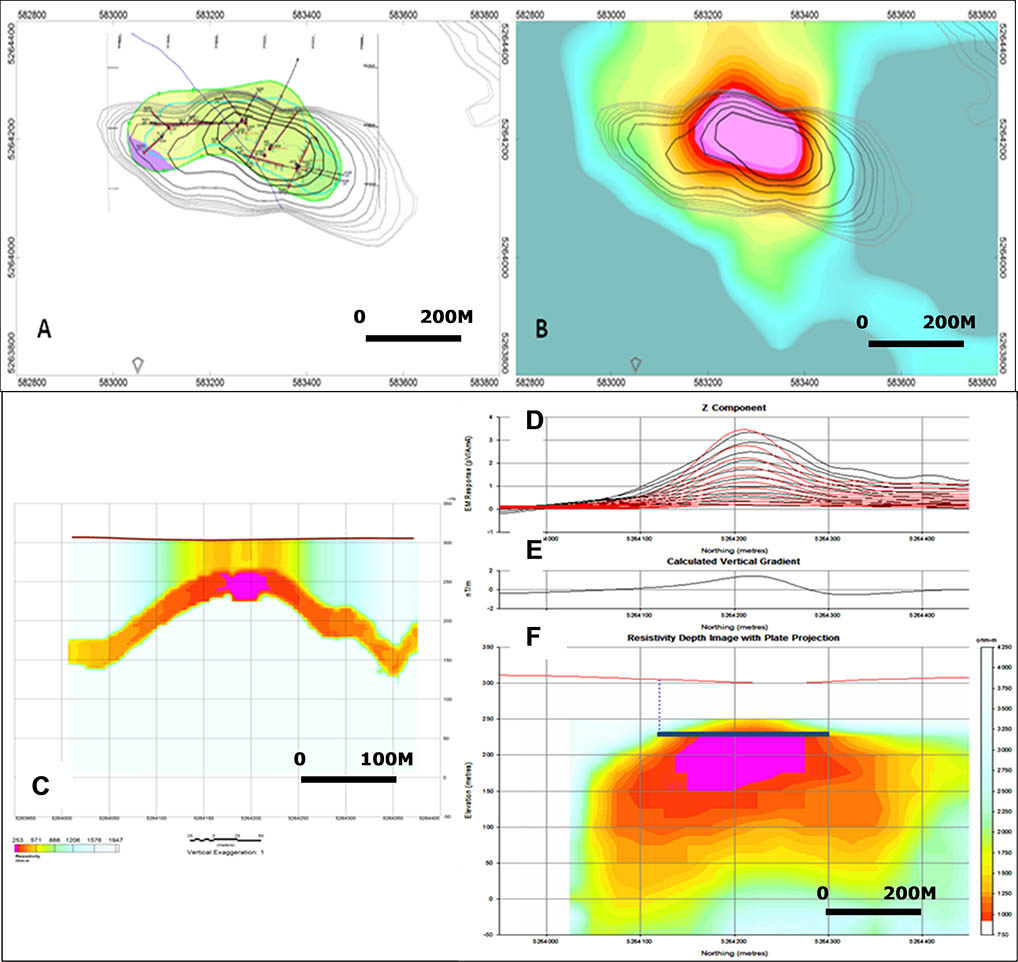
Figure 2a) Geological Outline of kimberlite pipe 95-2 in plan view with CVG contours and 2b) grid of VTEM™ early time gate (ch8 = 39-45 μsec dB/dT) with CVG contours (B) (CVG- calculated vertical gradient of magnetic field)); 2c) Laterally Constrained Inversion; 2d) dB/dt Z measured response in black and forward model response in red; 2e) Calculated vertical gradient of total magnetic intensity; and 2f) plate model and RDI section, all along the anomaly on north-south Line 1050.